Applying Population Genetics to Conservation Biology
Conservation biology increasingly relies on the understanding of genetic principles to inform strategies aimed at preserving biodiversity. Three key concepts—genetic diversity, effective population size, and gene flow—are pivotal in maintaining the resilience and adaptability of populations. Each of these concepts contributes to how we approach conservation challenges in different ecosystems.
Genetic diversity forms the foundation for populations to adapt to changing environmental conditions, disease pressures, and other stressors. Populations with high genetic diversity harbor a broad range of traits, increasing the likelihood that some individuals can survive and reproduce under new conditions. Such adaptability is crucial in the face of climate change, habitat fragmentation, and emerging diseases. Low genetic diversity, on the other hand, can lead to inbreeding depression, where the accumulation of deleterious recessive alleles reduces fitness and increases susceptibility to disease. This reduction in fitness can further shrink already endangered populations, setting them on a path toward extinction. For example, the genetic bottlenecks experienced by cheetahs and the inbreeding issues in Florida panthers illustrate the detrimental effects of reduced genetic diversity. Effective population size is a critical measure in conservation because it determines the rate at which genetic drift—the random loss of alleles—affects a population. Small effective population sizes increase the rate of genetic drift, leading to the rapid loss of genetic diversity and fixation of deleterious alleles. Populations with low Ne are at higher risk of inbreeding depression, reducing their adaptive potential. Maintaining an effective population size above critical thresholds is essential to ensure long-term genetic health and viability. Genetic management plans for endangered species, such as California condors and the Tasmanian devil, emphasize increasing Ne through captive breeding, managed introductions, and genetic rescue. Gene flow, or the transfer of genes between populations, plays a vital role in conservation biology by maintaining genetic diversity and reducing the risks associated with inbreeding. Connectivity between populations ensures that genes can move across the landscape, allowing populations to adapt and remain resilient. This connectivity counters genetic drift and introduces beneficial alleles into smaller populations. Barriers to gene flow, such as habitat fragmentation due to agriculture or urbanization, isolate populations, increasing their susceptibility to genetic issues. For instance, highways and other infrastructure often disrupt migration corridors for large mammals. In aquatic environments, dams can block fish migrations, leading to isolated populations and reducing genetic diversity. Restoring gene flow through wildlife corridors, habitat restoration, and assisted migration can help re-establish connectivity. Corridors enable the natural movement of individuals between fragmented habitats, while habitat restoration efforts create stepping stones that facilitate gene flow. Assisted migration moves individuals or entire populations to areas where they can thrive, particularly for species with limited dispersal capabilities.
Genetic Management of Endangered Species Using Captive Breeding Programs
Captive breeding programs for endangered species are critical tools for preventing extinction and maintaining biodiversity. They offer a controlled environment to support population growth, genetic management, and eventual reintroduction into the wild. To achieve these goals effectively, population genetic principles such as genetic diversity, effective population size, and gene flow are carefully incorporated into the design and management of these programs. The primary goal in captive breeding is to maximize and maintain genetic diversity, which ensures that the population retains a broad range of traits to adapt to environmental change.
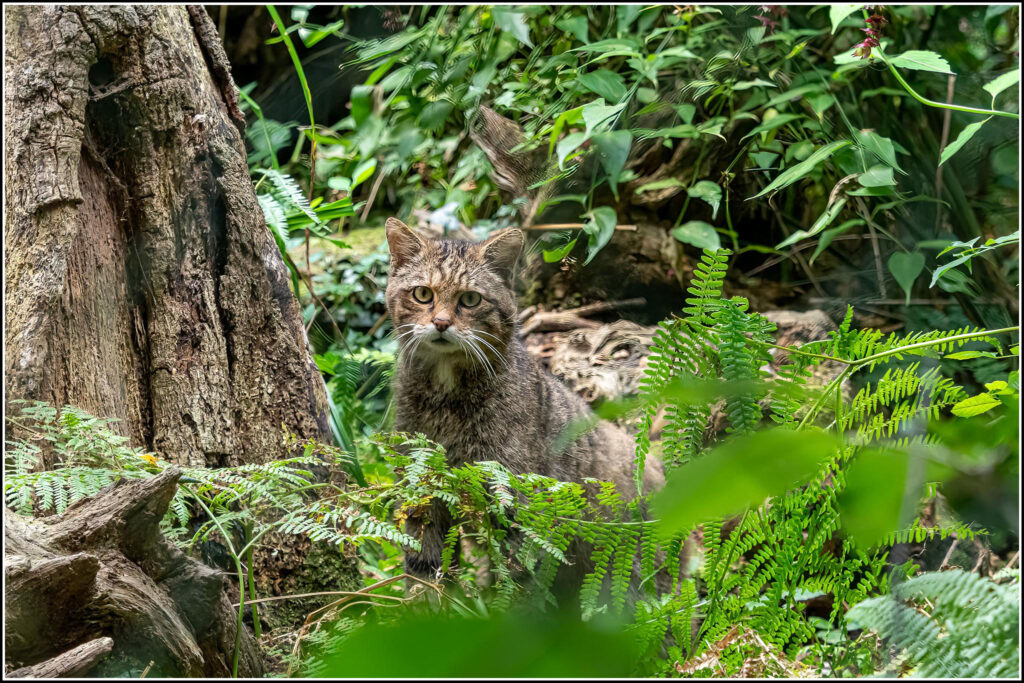
Captive breeding programs begin with a genetically diverse founding population, sourced from a wide range of wild individuals. This initial diversity forms the basis for long-term management, ensuring that as many alleles as possible are represented. Detailed records of breeding pairs, family histories, and individual genetic markers are then used to minimize inbreeding. By tracking pedigrees, managers avoid mating closely related individuals, thereby reducing inbreeding depression. The reproductive contribution of each founder’s lineage is balanced across generations. Breeding pairs are chosen to ensure that no particular genetic lineage dominates, preventing the loss of alleles due to genetic drift. Effective population size is managed to limit genetic drift and maintain genetic diversity over time. This involves pairing individuals strategically over time ensures that the genetic contributions are as equal as possible, preventing dominance by a few individuals and reducing skewed reproductive success (breeding rotation), monitoring generation intervals and life history traits allows for optimizing breeding schedules. By staggering the ages of breeding individuals, the effective population size is increased, slowing the loss of genetic variation. Finally, sufficient numbers of breeding individuals are maintained to keep the effective population size high. This often requires maintaining larger captive populations than the minimum needed for short-term survival.
Captive breeding programs face challenges, such as maintaining natural behaviors and avoiding domestication effects. Genetic management requires adaptive strategies that respond to new data, environmental changes, and emerging genetic issues. Managers must continuously assess genetic markers, monitor population health, and adjust breeding plans accordingly.
GROUP REFLECTION QUESTION
Media Attributions
- scottish wildcat © Smudge9000 is licensed under a CC BY-SA (Attribution ShareAlike) license