Early Molecular Markers
Allozymes – the first true marker
Allozymes are variants of enzymes encoded by different alleles of the same gene, and they can be detected through electrophoresis, a technique that separates charged molecules based on their size and charge. Allozyme analysis emerged as one of the earliest methods for studying genetic variation in natural populations. Before the advent of DNA sequencing technologies, scientists relied on protein-based markers, such as allozymes, to study genetic diversity and population structure. The principle behind allozyme analysis is based on the fact that different alleles of a gene may produce enzymes with slightly different amino acid sequences, resulting in variations in enzyme mobility during electrophoresis. These differences can be visualized as distinct bands on a gel, allowing researchers to infer genetic variation within and between populations.
Methodology:
- Sample Collection and Preparation: Researchers collect tissue samples (e.g., blood, muscle, or plant tissue) from individuals within a population.
- Protein Extraction: Proteins are extracted from the tissue samples using biochemical methods.
- Electrophoresis: The extracted proteins are separated based on their charge and size using electrophoresis. This involves applying an electric current to a gel matrix containing the protein samples. Proteins migrate through the gel at different rates based on their charge and size, resulting in distinct bands corresponding to different allozyme variants.
- Staining and Visualization: After electrophoresis, the gel is stained to visualize the allozyme bands. Each band represents a different allozyme variant encoded by different alleles of the same gene.
- Analysis: Researchers analyze the allozyme banding patterns to estimate genetic diversity, population structure, gene flow, and evolutionary relationships among populations.
Allozyme markers have been widely used in molecular ecology to address various research questions, including (1) assessing genetic diversity within populations, (2) investigating population structure and genetic differentiation, (3) estimating gene flow and migration patterns, (4) studying evolutionary relationships and phylogenetic reconstruction and (5) monitoring genetic responses to environmental changes and anthropogenic impacts. Despite being an older technology, allozyme analysis laid the groundwork for modern molecular ecology and provided valuable insights into the genetic diversity and evolutionary dynamics of natural populations. While it has been largely replaced by DNA-based markers such as microsatellites and SNPs due to their higher resolution and ease of analysis, allozyme analysis remains relevant in certain contexts and continues to contribute to our understanding of biodiversity and conservation biology.
Limitations of Allozymes
Allozyme analysis, while a valuable tool in molecular ecology and evolutionary biology, has several limitations that researchers should consider. Firstly, allozyme analysis provides relatively low resolution compared to newer DNA-based markers like microsatellites and SNPs. This is because allozymes are the products of gene expression rather than the genes themselves, and they may not fully capture the underlying genetic variation present in a population. Secondly, allozyme analysis typically examines a limited number of enzyme loci, often ranging from a few to several dozen loci. This limited number of markers may not provide sufficient coverage of the genome to accurately capture genetic diversity and population structure, especially in species with large or complex genomes. Moreover, allozymes are subject to genetic homoplasy, where different alleles at different loci may produce the same allozyme phenotype. This can lead to misleading interpretations of genetic relationships and population structure, particularly when using a small number of allozyme loci. Furthermore, allozyme analysis requires specialized laboratory techniques for protein extraction, electrophoresis, and staining, which can be time-consuming, labor-intensive, and technically challenging. Additionally, obtaining high-quality allozyme data may require optimization of experimental conditions and protocols. Additionally, allozyme expression can be influenced by environmental factors such as temperature, pH, and substrate availability. Variation in environmental conditions among sampling locations may confound allozyme patterns and complicate interpretations of genetic diversity and population structure. Lastly, allozyme analysis focuses on variations in protein-coding genes and may not capture genetic variation in non-coding regions of the genome, which can play important roles in evolution and adaptation.
Restriction Fragment Length Polymorphism (RFLP)
RFLPs are variations in DNA sequences that result in differences in the lengths of DNA fragments when cut with restriction enzymes. These variations occur due to differences in the recognition sites of restriction enzymes among individuals or populations. In RFLP analysis, DNA samples are first digested with restriction enzymes, which cut the DNA at specific recognition sites. The resulting DNA fragments are then separated by size using gel electrophoresis. Because individuals may have different alleles with different restriction sites, the resulting banding patterns on the gel can reveal genetic variation within and between populations. RFLPs were one of the earliest types of molecular markers used in genetics and have been widely applied in population genetics, phylogenetics, and forensic science. They have been used to study genetic diversity, population structure, evolutionary relationships, and gene mapping in various organisms.
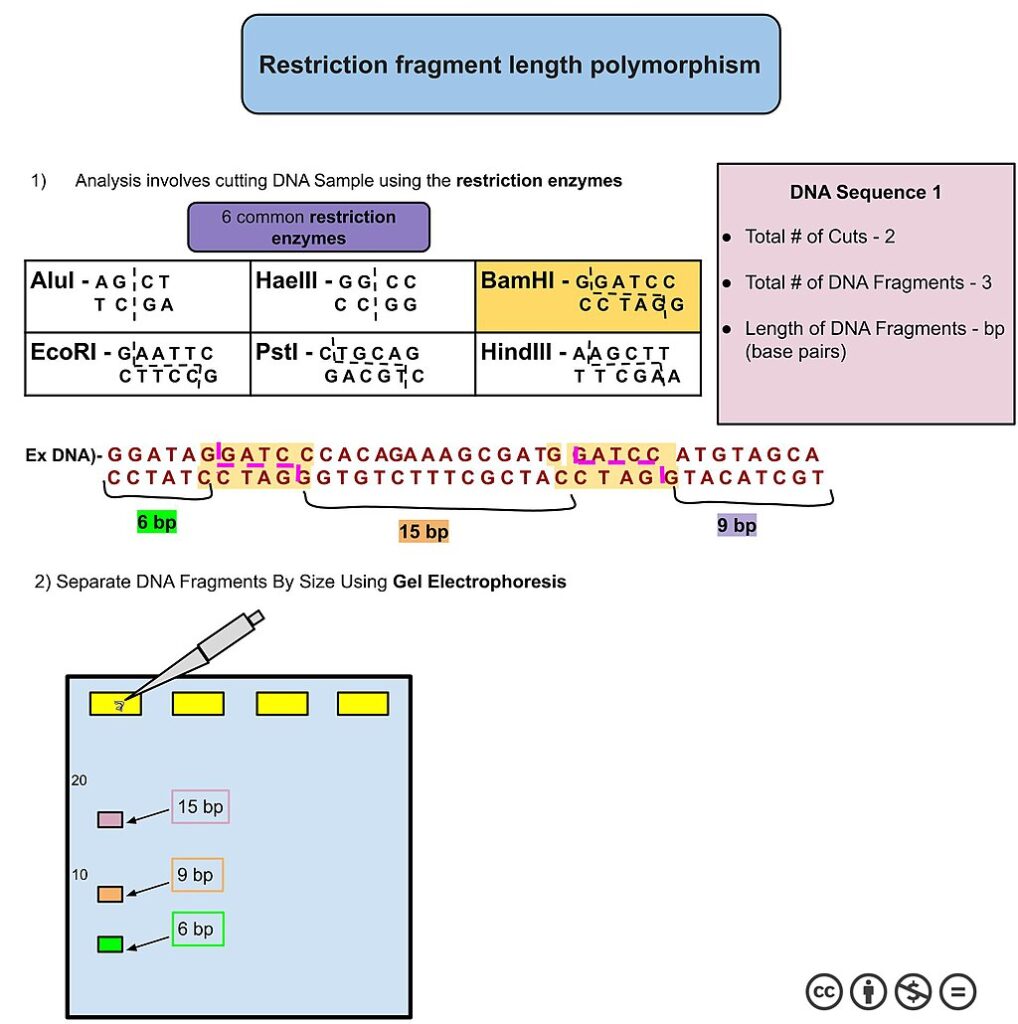
However, RFLP analysis has several limitations:
- Low Throughput: RFLP analysis typically examines a limited number of restriction sites, and the process can be time-consuming and labor-intensive, especially when analyzing multiple loci or large sample sizes.
- Technically Challenging: RFLP analysis requires specialized laboratory techniques for DNA extraction, restriction enzyme digestion, gel electrophoresis, and staining, which can be technically challenging and prone to error.
- Limited Resolution: RFLPs may have lower resolution compared to newer molecular markers such as microsatellites and SNPs. The size differences between DNA fragments may be relatively small, leading to overlapping bands on the gel and difficulty in accurately determining fragment sizes.
- Inability to Detect Point Mutations: RFLPs are not suitable for detecting single nucleotide polymorphisms (SNPs) or point mutations, as they primarily detect variations in DNA fragment length resulting from differences in restriction enzyme recognition sites.
- Limited Information Content: RFLPs provide limited information about the genetic variation within a population compared to DNA sequence data. They may not capture variation in non-coding regions of the genome or provide insights into gene function or gene regulation.
Despite these limitations, RFLP analysis has been instrumental in early genetic studies and has contributed valuable insights into genetic diversity and population structure in various organisms. However, it has largely been supplanted by more advanced molecular markers with higher throughput and resolution, such as microsatellites, SNPs, and next-generation sequencing technologies.
THINK/PAIR/SHARE
1. Given that both allozyme and RFLP analyses have lower resolution compared to modern DNA-based markers like microsatellites and SNPs, discuss the potential scenarios or research contexts where these older techniques might still be advantageous or necessary. What are the trade-offs involved in choosing these methods over newer technologies?
Media Attributions
- 1024px-Restriction_Fragment_Length_Polymorphism © Lolyas39 is licensed under a CC BY-SA (Attribution ShareAlike) license