Genome Organization
The genomes of living organisms, whether prokaryotic or eukaryotic, are the fundamental blueprints that dictate their biological functions and evolutionary potential. The organization of these genomes reflects the different structural and functional requirements of the two groups, influencing their biological complexity, adaptability, and ecological interactions.
Prokaryotic Genomes
Prokaryotic genomes are generally simpler in structure compared to their eukaryotic counterparts. Prokaryotes, which include bacteria and archaea, typically have a single, circular chromosome. This chromosome is located in the nucleoid, a region within the cell that is not membrane-bound. The circular nature of the chromosome is advantageous, as it allows for continuous replication. Additionally, many prokaryotes possess plasmids, which are small, circular DNA molecules that replicate independently of the chromosomal DNA. These plasmids often carry genes that confer advantageous traits, such as antibiotic resistance, facilitating rapid adaptation to changing environments. The genome size of prokaryotes tends to be much smaller than that of eukaryotes, reflecting their generally less complex biological processes. Prokaryotic genomes are densely packed with genes, and intergenic regions are minimal, resulting in a high gene density. This efficient organization reflects the streamlined nature of prokaryotic genomes, which often lack introns, making their genes continuous coding sequences. This arrangement allows for efficient transcription and translation, facilitating rapid cellular responses to environmental stimuli.
Eukaryotic Genomes
Eukaryotic genomes are more complex and varied in their structure. Eukaryotes, which include animals, plants, fungi, and protists, typically have multiple linear chromosomes contained within a membrane-bound nucleus. This structural compartmentalization allows for greater regulation of gene expression and more complex interactions between genetic elements. Eukaryotic chromosomes are associated with histone proteins, which help package the DNA into a compact, organized structure known as chromatin. The chromatin structure plays a crucial role in regulating gene expression, as regions of tightly packed chromatin (heterochromatin) are generally transcriptionally inactive, while loosely packed chromatin (euchromatin) is transcriptionally active.
Eukaryotic genomes are significantly larger than prokaryotic genomes, often containing a vast amount of non-coding DNA. This non-coding DNA includes introns, which are non-coding regions within genes that are spliced out during RNA processing, as well as various types of repetitive elements. The presence of introns allows for alternative splicing, a process that enables a single gene to produce multiple protein variants, increasing the functional diversity of the proteome. The repetitive elements, which include transposable elements and tandem repeats, contribute to genomic diversity and evolution through mechanisms such as gene duplication and genome rearrangement.
One of the key distinctions between prokaryotic and eukaryotic genomes lies in the regulation of gene expression. In prokaryotes, gene expression is typically regulated at the transcriptional level through mechanisms such as operons, where a single promoter controls the expression of multiple genes. This allows for coordinated expression of genes involved in related functions. In eukaryotes, gene regulation is more complex, involving multiple levels of control, including chromatin remodeling, transcriptional regulation, RNA processing, and post-transcriptional regulation. This complexity allows for precise spatial and temporal control of gene expression, enabling the development and differentiation of multicellular organisms. Another important aspect of genome organization is the presence of organellar genomes in eukaryotes. Eukaryotic cells contain mitochondria and, in the case of plants and algae, chloroplasts, both of which have their own genomes. These organellar genomes are typically circular and resemble prokaryotic genomes, reflecting their evolutionary origin from endosymbiotic bacteria. The organellar genomes encode essential components for energy production, and their presence adds an additional layer of genetic complexity to eukaryotic cells.
An Introduction to Mitochondrial DNA (mtDNA)
Mitochondrial DNA (mtDNA) is a type of extranuclear DNA located in the mitochondria, the energy-producing organelles within eukaryotic cells. Unlike nuclear DNA, which is inherited from both parents, mtDNA is typically maternally inherited, making it a valuable tool for tracing lineage and evolutionary patterns. However, using mtDNA to infer evolutionary relationships has both advantages and disadvantages. Most of the studies we will be exploring in this course will focus heavily on mtDNA and for lab, you will be amplifying a mitochondrial DNA gene-fragment. It is important that you understand the shortcomings of using this type of DNA for inferring evolutionary patterns.
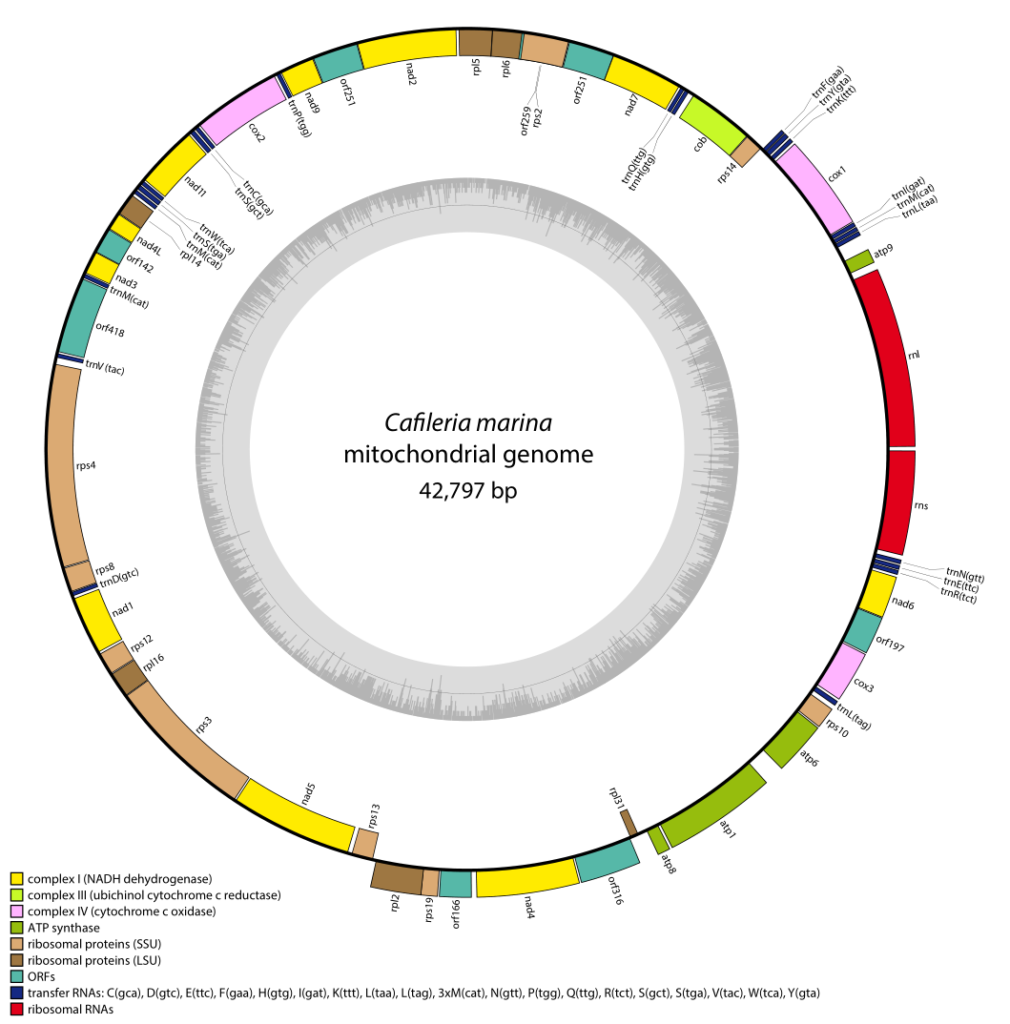
Advantages of using mtDNA in molecular ecology research
- Maternal Inheritance:
-
- mtDNA is passed down from the mother without recombination, allowing for clear lineage tracing.
- This feature is particularly useful for studying maternal lineages and resolving evolutionary relationships within species and closely related species.
- High Mutation Rate:
-
- mtDNA generally has a higher mutation rate compared to nuclear DNA.
- This characteristic provides greater genetic diversity over shorter evolutionary time scales, making it ideal for studying recent evolutionary events.
- Conserved Structure:
-
- The structure of mtDNA is relatively conserved across species, allowing for easy comparison of homologous regions.
- The consistent gene arrangement simplifies comparative studies and phylogenetic analyses.
- Abundance:
-
- Mitochondria contain multiple copies of mtDNA, which makes it easier to extract and amplify compared to nuclear DNA.
- This abundance is especially advantageous when dealing with degraded or ancient DNA samples, as often found in paleogenomics and forensic studies.
- Lack of Recombination:
-
- The absence of recombination in mtDNA provides a straightforward inheritance pattern, which simplifies the interpretation of evolutionary relationships.
Disadvantages of using mtDNA for molecular ecology research
- Maternal Inheritance:
-
- While maternal inheritance is useful for studying maternal lineages, it provides a biased view of the evolutionary history of an organism.
- It ignores the paternal lineage and provides no information about recombination-based evolution or nuclear DNA contributions.
- High Mutation Rate:
-
- The high mutation rate can lead to homoplasy, where unrelated lineages independently acquire similar mutations.
- Homoplasy can obscure true evolutionary relationships and complicate phylogenetic analyses.
- Limited Genomic Information:
-
- The mitochondrial genome is small and contains limited genetic information compared to the nuclear genome.
- This limitation restricts the amount of evolutionary data available for analysis and may not reflect the broader evolutionary patterns present in the nuclear genome.
- Selective Sweeps and Bottlenecks:
-
- mtDNA is subject to selective sweeps and genetic bottlenecks, which can reduce genetic diversity and obscure evolutionary history.
- These events can result in misleading interpretations of population history and evolutionary relationships.
- Nuclear-Mitochondrial DNA (Numts):
-
- Nuclear copies of mitochondrial DNA, known as NUMTs, can complicate analyses by producing misleading sequences that resemble true mtDNA.
- The presence of Numts requires careful interpretation of sequencing data to avoid incorrect phylogenetic inferences.
Media Attributions
- Cafileria_marina_mitogenome © Description English: Mitochondrial genome of Cafileria marina (from the article's Supplemental Material). Date 26 June 2019 Source (2019). "Morphology, Ultrastructure, and Mitochondrial Genome of the Marine Non-Photosynthetic Bicosoecid Cafileria marina Gen. et sp. nov.". Microorganisms 7 (8): 240. DOI:10.3390/microorganisms7080240. Author Dagmar Jirsová, Zoltán Füssy, Jitka Richtová, Ansgar Gruber and Miroslav Oborník is licensed under a CC BY (Attribution) license