The Extinction Vortex
What is an extinction vortex?
The extinction vortex is a self-reinforcing process in which small populations are increasingly likely to decline toward extinction due to a combination of genetic, demographic, and environmental factors. As a population diminishes, it becomes increasingly vulnerable to genetic and ecological challenges, creating a feedback loop that accelerates its path toward extinction. This concept was first formally described by conservation biologist Michael Soulé in the 1980s. He categorized the factors contributing to the extinction vortex into two major categories: deterministic factors (such as habitat destruction and overhunting) and stochastic factors (random events like natural disasters, disease outbreaks, or genetic issues). Together, these factors amplify each other’s effects.
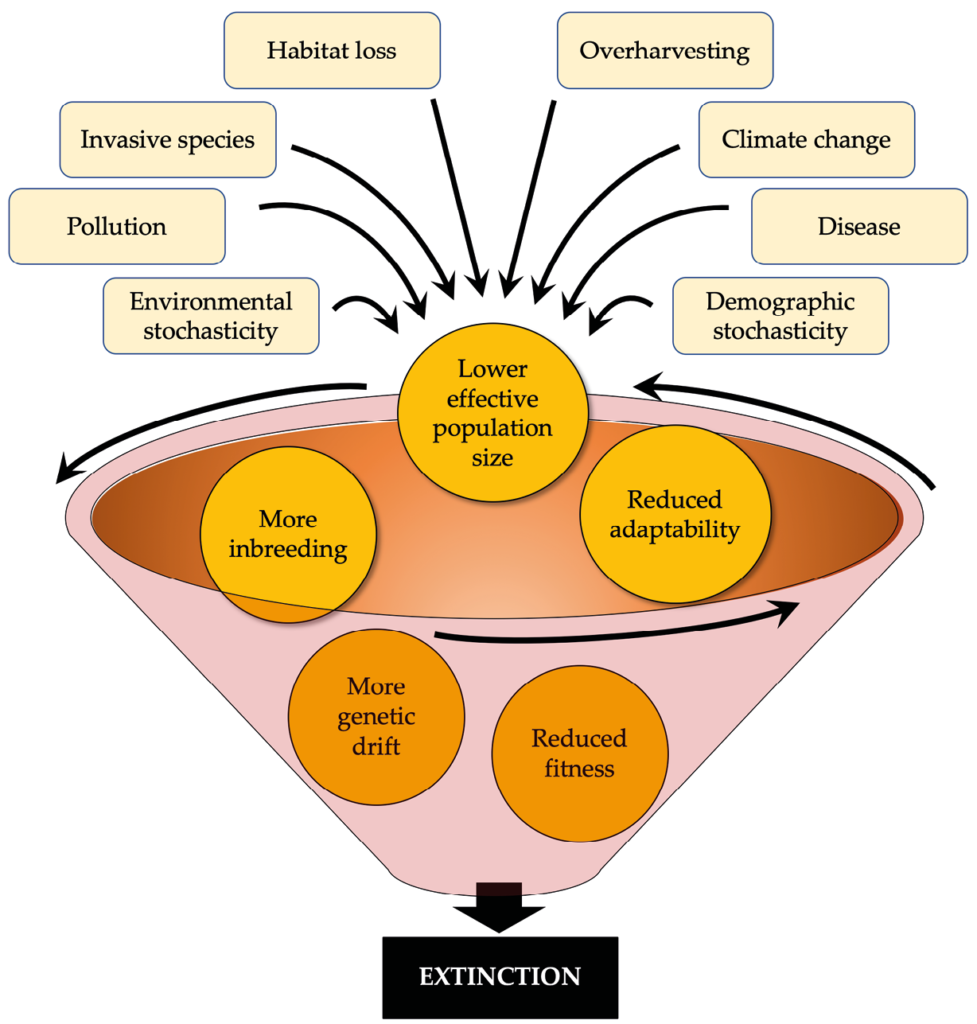
Genetic factors play a significant role in driving the extinction vortex. Two critical concepts in this regard are genetic load and inbreeding depression:
- Genetic Load
Genetic load refers to the accumulation of deleterious alleles in a population’s gene pool, reducing the overall fitness of individuals. In large populations, selection can effectively eliminate harmful alleles. However, in small populations, genetic drift—a random change in allele frequencies—may cause these alleles to become more frequent, increasing the genetic load. This results in a general decline in population fitness, making individuals more susceptible to disease and reducing reproductive success. - Inbreeding Depression
As a population shrinks, the likelihood of close relatives breeding increases, leading to inbreeding. Inbreeding depression describes the reduced biological fitness observed in offspring due to the increased expression of recessive deleterious alleles. This results in individuals with lower fertility, higher mortality rates, and greater susceptibility to disease, further reducing the population’s ability to recover.
Demographic and Environmental Impacts
Genetic problems are compounded by demographic and environmental challenges that further weaken populations such as demographic stochasticity, whereby small populations are disproportionately affected by random fluctuations in birth and death rates. This demographic stochasticity can lead to skewed sex ratios and poor recruitment, accelerating the decline. In environmental stochasticity, small populations are highly vulnerable to environmental changes, such as droughts, floods, and temperature fluctuations and a single catastrophic event can cause local extinction in small, isolated groups. Finally, reduced population sizes also lead to Allee effects, where individuals struggle to find mates or cooperate effectively. This reduces reproduction and survival rates.
The Feedback Loop
The extinction vortex arises because these genetic, demographic, and environmental factors form a feedback loop that worsens over time. As genetic diversity declines, inbreeding depression increases, which, coupled with demographic and environmental stochasticity, reduces reproductive success and survival rates. This further shrinks the population size, exacerbating inbreeding and the genetic load, and making the population increasingly vulnerable to stochastic events.
Breaking the Vortex
Breaking the extinction vortex requires intensive conservation efforts to restore populations above critical sizes. Strategies include:
- Genetic Management: Introducing new genetic material through translocation or managed breeding can reduce genetic load and inbreeding depression.
- Habitat Restoration: Improving habitats and creating wildlife corridors can reduce isolation and promote natural gene flow.
- Captive Breeding and Reintroduction: Establishing ex-situ populations can help maintain genetic diversity and serve as a source for reintroducing individuals into the wild.
Genetic purging is an intriguing concept in conservation genetics that suggests populations can, under certain conditions, rid themselves of deleterious alleles and recover from the brink of an extinction vortex. This process operates within small, isolated populations where the effects of inbreeding and genetic drift are pronounced. In the context of genetic purging, the increased inbreeding that typically contributes to inbreeding depression can paradoxically become a mechanism for recovery. As inbreeding intensifies in a small population, deleterious recessive alleles are more frequently expressed due to the pairing of similar genes. This leads to individuals with harmful traits manifesting these traits more clearly, which often results in reduced survival or reproductive success. When these individuals with detrimental traits have lower fitness and fail to reproduce as effectively, the harmful alleles they carry are less likely to be passed on to the next generation. Over time, this can lead to a reduction in the population’s genetic load — the collective burden of these harmful alleles. The critical factor here is the balance between the speed at which deleterious alleles are purged through natural selection and the rate at which genetic diversity is lost due to genetic drift and inbreeding.
For genetic purging to be beneficial, a population must have enough genetic diversity to survive the initial increase in inbreeding depression. If a population is too small or its genetic diversity is too low, the immediate negative impacts of inbreeding depression might overwhelm any potential long-term benefits from purging. Thus, while genetic purging can help a population recover, it is not a panacea and works within specific limits. Natural examples of genetic purging are rare but insightful. Some conservationists observe this process in captive breeding programs where the initial generations suffer high levels of inbreeding depression, but later generations show signs of recovery as harmful alleles are eliminated. This suggests that careful management, even of very small populations, can harness the purging process to improve population viability over time.
However, relying solely on genetic purging is risky. Conservation efforts often complement potential purging with strategies like introducing new genetic material to boost diversity, establishing protected areas to support larger, more stable populations, and enhancing habitat connectivity to facilitate gene flow. These measures ensure that while purging may aid recovery, it is part of a broader strategy to restore and sustain biodiversity. In essence, genetic purging is a nuanced process where the detrimental effects of inbreeding can lead to a long-term reduction in genetic load, potentially aiding population recovery. Yet, this process requires careful consideration and often needs to be supported by proactive conservation measures to ensure the overall health and resilience of endangered populations.
Media Attributions
- The_extinction_vortex_describes_a_process_whereby_the_factors_that_affect_small_populations_can_drive_its_size_progressively_downward_towards_extinction © John W. Wilson, Richard B. Primack is licensed under a CC BY (Attribution) license